Active transport, a crucial process in biology, involves moving molecules across a cell membrane against their concentration gradient. Are you curious about how this process works and its significance? At WHAT.EDU.VN, we provide clear and comprehensive explanations to help you understand complex scientific concepts. Learn about the different types of active transport, its applications, and how it differs from passive transport. Explore electrochemical gradients and ATP hydrolysis explained simply.
1. Understanding Active Transport: The Basics
Active transport is a biological process that moves molecules and ions across cell membranes. Unlike passive transport, which doesn’t require energy, active transport necessitates cellular energy to move substances against their concentration gradient, from an area of lower concentration to an area of higher concentration. This is essential for maintaining the right balance of substances inside cells and carrying out various biological functions.
1.1. Why is Active Transport Necessary?
Cells need active transport to maintain internal conditions that differ from their surroundings. This includes:
- Nutrient uptake: Cells actively transport essential nutrients, such as glucose and amino acids, even when their concentration is lower outside the cell.
- Waste removal: Cells remove waste products, even if their concentration is higher outside the cell.
- Ion balance: Cells maintain specific ion concentrations, like sodium, potassium, and calcium, which are crucial for nerve function, muscle contraction, and other processes.
1.2. Active Transport vs. Passive Transport
Feature | Active Transport | Passive Transport |
---|---|---|
Energy Required | Yes (ATP or electrochemical gradient) | No |
Gradient Direction | Against concentration gradient (low to high) | Along concentration gradient (high to low) |
Examples | Sodium-potassium pump, glucose uptake in intestines | Diffusion of oxygen in lungs, osmosis of water molecules |


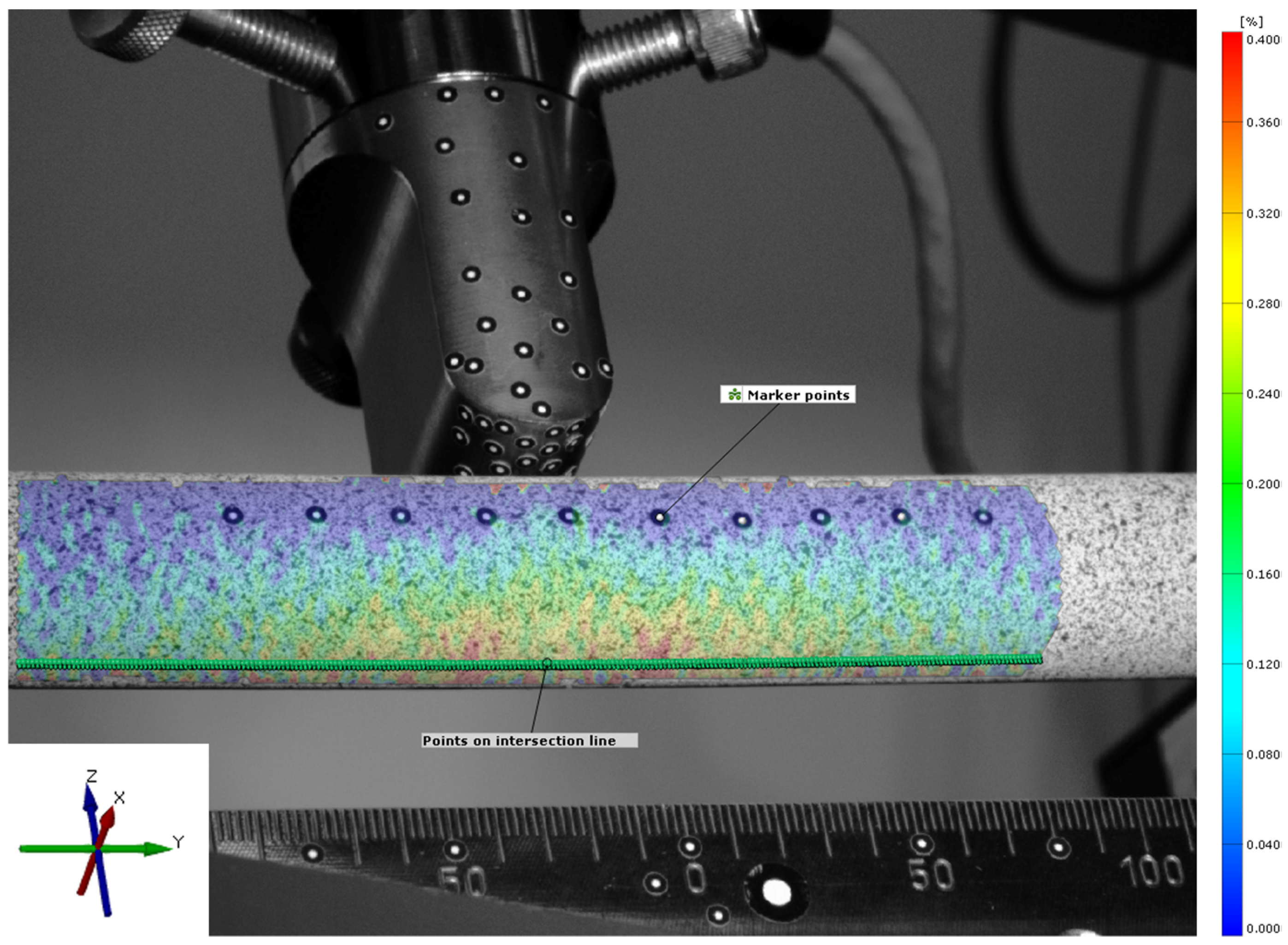
1.3. Types of Active Transport
Active transport is broadly classified into two main types:
- Primary Active Transport: Uses chemical energy, typically in the form of ATP, to directly move molecules across the membrane.
- Secondary Active Transport: Uses the electrochemical gradient created by primary active transport to move other molecules. It does not directly use ATP.
2. Primary Active Transport: Direct Energy Use
Primary active transport directly uses ATP hydrolysis to move molecules against their concentration gradient. This process involves specific transmembrane proteins that act as pumps.
2.1. How Primary Active Transport Works
- Binding: The molecule to be transported binds to the protein pump.
- ATP Hydrolysis: ATP is hydrolyzed (broken down) into ADP (adenosine diphosphate) and inorganic phosphate, releasing energy.
- Conformational Change: The energy released causes the protein pump to change shape.
- Translocation: The molecule is moved across the membrane.
- Release: The molecule is released on the other side of the membrane, and the pump returns to its original shape.
2.2. Key Examples of Primary Active Transport
2.2.1. Sodium-Potassium Pump (Na+/K+ ATPase)
The sodium-potassium pump is found in the plasma membrane of most animal cells. It maintains the electrochemical gradient by transporting three sodium ions (Na+) out of the cell and two potassium ions (K+) into the cell, both against their concentration gradients. This process requires ATP.
Importance:
- Nerve impulse transmission: Essential for maintaining the resting membrane potential in nerve cells.
- Muscle contraction: Plays a crucial role in muscle cell function.
- Osmotic balance: Helps regulate cell volume by controlling ion concentrations.
2.2.2. Calcium Pump (Ca2+ ATPase)
The calcium pump transports calcium ions (Ca2+) out of the cell or into the endoplasmic reticulum (ER) in muscle cells. This helps maintain low calcium concentrations in the cytoplasm, which is crucial for various cellular processes.
Importance:
- Muscle contraction: Regulates muscle contraction and relaxation.
- Signal transduction: Involved in cell signaling pathways.
- Neurotransmitter release: Controls the release of neurotransmitters at synapses.
2.2.3. Hydrogen-Potassium Pump (H+/K+ ATPase)
Found in the parietal cells of the stomach lining, the hydrogen-potassium pump secretes hydrogen ions (H+) into the stomach lumen, making the stomach acidic. This is essential for digestion.
Importance:
- Digestion: Aids in the breakdown of food in the stomach.
- Protection: Kills bacteria and other pathogens in the stomach.
2.3. How ATP Powers Active Transport
ATP (adenosine triphosphate) is the primary energy currency of cells. When ATP is hydrolyzed, it releases energy that the protein pump uses to change shape and move molecules across the membrane.
ATP Hydrolysis Equation:
ATP + H2O → ADP + Pi + Energy
Where:
- ATP = Adenosine Triphosphate
- H2O = Water
- ADP = Adenosine Diphosphate
- Pi = Inorganic Phosphate
- Energy = Energy released for active transport
3. Secondary Active Transport: Harnessing Electrochemical Gradients
Secondary active transport does not directly use ATP. Instead, it uses the electrochemical gradient created by primary active transport. This gradient provides the energy needed to move other molecules across the membrane.
3.1. How Secondary Active Transport Works
- Primary Transport Creates Gradient: Primary active transport establishes an electrochemical gradient, typically of sodium ions (Na+).
- Coupling: Another molecule (e.g., glucose, amino acid) binds to the transport protein along with the ion moving down its concentration gradient.
- Co-transport: The energy released by the ion moving down its gradient drives the transport of the other molecule against its gradient.
3.2. Types of Secondary Active Transport
3.2.1. Symport (Co-transport)
In symport, both the ion and the other molecule move in the same direction across the membrane.
Example: Sodium-Glucose Co-transporter (SGLT)
The sodium-glucose co-transporter (SGLT) in the small intestine and kidney transports glucose into the cell along with sodium ions. The sodium gradient, established by the sodium-potassium pump, provides the energy for glucose to move against its concentration gradient.
Importance:
- Glucose absorption: Enables glucose absorption in the intestines and kidneys.
- Nutrient uptake: Facilitates the uptake of other nutrients and molecules.
3.2.2. Antiport (Exchange)
In antiport, the ion and the other molecule move in opposite directions across the membrane.
Example: Sodium-Calcium Exchanger (NCX)
The sodium-calcium exchanger (NCX) in heart muscle cells transports sodium ions into the cell and calcium ions out of the cell. The sodium gradient provides the energy for calcium to move against its concentration gradient.
Importance:
- Calcium regulation: Helps maintain low calcium concentrations in the cytoplasm, preventing excessive muscle contraction.
- Cardiac function: Crucial for regulating heart muscle contraction and relaxation.
3.3. Importance of Electrochemical Gradients
Electrochemical gradients are essential for secondary active transport. These gradients are created by primary active transport and store potential energy that can be used to drive the movement of other molecules.
Components of Electrochemical Gradients:
- Concentration Gradient: The difference in concentration of a substance across the membrane.
- Electrical Gradient: The difference in electrical charge across the membrane.
Together, these gradients create an electrochemical potential that drives the movement of ions and other molecules.
4. Examples of Active Transport in Biological Systems
Active transport plays a vital role in various biological systems, ensuring cells maintain the necessary internal conditions for proper function.
4.1. Active Transport in the Kidneys
In the kidneys, active transport is crucial for reabsorbing essential substances from the filtrate back into the bloodstream.
- Glucose reabsorption: SGLT proteins reabsorb glucose from the filtrate in the proximal tubules.
- Amino acid reabsorption: Various active transport proteins reabsorb amino acids.
- Ion balance: Sodium-potassium pumps and other active transport proteins maintain ion balance in the kidneys.
4.2. Active Transport in the Nervous System
In the nervous system, active transport is essential for maintaining the resting membrane potential and transmitting nerve impulses.
- Sodium-potassium pump: Maintains the resting membrane potential in nerve cells.
- Calcium pump: Regulates calcium levels in nerve cells, controlling neurotransmitter release.
- Neurotransmitter reuptake: Active transport proteins reabsorb neurotransmitters from the synapse, regulating signal transmission.
4.3. Active Transport in the Digestive System
In the digestive system, active transport is crucial for absorbing nutrients from the small intestine into the bloodstream.
- Glucose absorption: SGLT proteins transport glucose from the intestinal lumen into the epithelial cells.
- Amino acid absorption: Various active transport proteins absorb amino acids.
- Ion absorption: Active transport proteins regulate the absorption of ions like sodium, potassium, and chloride.
5. Clinical Significance of Active Transport
Dysfunction in active transport mechanisms can lead to various diseases and medical conditions.
5.1. Cystic Fibrosis
Cystic fibrosis (CF) is a genetic disorder caused by a mutation in the CFTR (cystic fibrosis transmembrane conductance regulator) gene. This gene encodes a chloride channel that uses ATP to transport chloride ions across cell membranes.
Impact on Active Transport:
- The mutated CFTR protein cannot properly transport chloride ions, leading to thick mucus buildup in the lungs, pancreas, and other organs.
- This affects the movement of sodium and water, disrupting the hydration of mucosal surfaces.
Clinical Manifestations:
- Recurrent lung infections
- Pancreatic insufficiency
- Malabsorption
- Steatorrhea (fatty stools)
5.2. Renal Tubular Acidosis (RTA)
Renal tubular acidosis (RTA) is a condition characterized by the kidneys’ inability to properly acidify the urine.
Impact on Active Transport:
- Type I RTA (distal RTA) involves impaired active transport of hydrogen ions (H+) into the urine due to defects in H+/K+ ATPases.
- This leads to increased urinary alkalinity and potential kidney stone formation.
Clinical Manifestations:
- Metabolic acidosis
- Kidney stones
- Bone disease
5.3. Bartter Syndrome
Bartter syndrome is a genetic disorder characterized by defects in the sodium-potassium-chloride co-transporter (NKCC) in the kidneys.
Impact on Active Transport:
- The defective NKCC protein impairs the reabsorption of sodium, potassium, and chloride ions in the kidneys.
- This leads to hypokalemia (low potassium levels) and metabolic alkalosis.
Clinical Manifestations:
- Muscle weakness
- Fatigue
- Increased urination
- Electrolyte imbalances
5.4. Digoxin and Heart Failure
Digoxin is a cardiac glycoside used to treat heart failure. It works by inhibiting the sodium-potassium pump in cardiac cells.
Impact on Active Transport:
- Inhibition of the sodium-potassium pump leads to increased intracellular sodium levels.
- This inhibits the sodium-calcium exchanger, resulting in increased intracellular calcium levels.
- Increased calcium levels enhance cardiac contractility (positive inotropy), improving heart function.
Clinical Use:
- Treatment of heart failure to improve cardiac output and reduce symptoms.
6. Active Transport in Drug Delivery
Active transport is also harnessed in drug delivery systems to enhance the uptake of drugs into specific cells or tissues.
6.1. Targeted Drug Delivery
- Drugs can be designed to bind to specific transport proteins on target cells, such as cancer cells.
- These transport proteins then actively transport the drug into the cell, increasing its effectiveness.
6.2. Nanoparticles and Active Transport
- Nanoparticles can be engineered to mimic molecules that are actively transported into cells.
- This allows the nanoparticles to be selectively taken up by target cells, delivering drugs or imaging agents directly to the site of action.
7. Frequently Asked Questions (FAQs) About Active Transport
To further clarify your understanding of active transport, here are some frequently asked questions:
Question | Answer |
---|---|
What is the main difference between active and passive transport? | Active transport requires energy (ATP or electrochemical gradient) to move molecules against their concentration gradient, while passive transport does not require energy and moves molecules down their concentration gradient. |
What are the two main types of active transport? | Primary active transport, which directly uses ATP, and secondary active transport, which uses the electrochemical gradient created by primary active transport. |
How does the sodium-potassium pump work? | The sodium-potassium pump uses ATP to transport three sodium ions (Na+) out of the cell and two potassium ions (K+) into the cell, maintaining the electrochemical gradient. |
What is the role of active transport in the kidneys? | Active transport in the kidneys is crucial for reabsorbing essential substances like glucose, amino acids, and ions from the filtrate back into the bloodstream. |
How does cystic fibrosis affect active transport? | Cystic fibrosis is caused by a mutation in the CFTR gene, which encodes a chloride channel that uses ATP to transport chloride ions. The mutated protein disrupts chloride transport, leading to thick mucus buildup. |
What is secondary active transport? | Secondary active transport uses the electrochemical gradient created by primary active transport to move other molecules across the membrane. It does not directly use ATP. |
What is the difference between symport and antiport? | In symport, both the ion and the other molecule move in the same direction, while in antiport, they move in opposite directions. |
How does active transport contribute to drug delivery? | Active transport can be harnessed to deliver drugs more effectively to specific cells or tissues by targeting transport proteins on those cells. |
What is ATP, and why is it important in active transport? | ATP (adenosine triphosphate) is the primary energy currency of cells. It provides the energy needed for primary active transport proteins to change shape and move molecules against their concentration gradient. |
Can active transport be affected by diseases? | Yes, several diseases, such as cystic fibrosis, renal tubular acidosis, and Bartter syndrome, are caused by defects in active transport mechanisms. |
8. Conclusion: The Importance of Active Transport
Active transport is a fundamental process in biology, essential for maintaining cellular homeostasis, nutrient uptake, waste removal, and various other functions. Understanding the mechanisms and examples of active transport is crucial for comprehending how cells and biological systems work.
If you have more questions about active transport or any other scientific topic, don’t hesitate to ask on WHAT.EDU.VN. Our platform provides free and quick answers to all your queries. Our team of experts is here to help you explore the fascinating world of science.
Ready to Learn More?
Do you have burning questions about biology, chemistry, or any other subject? Don’t struggle alone. Visit WHAT.EDU.VN today and ask your question for free. Our community of experts is ready to provide quick, accurate, and easy-to-understand answers.
Address: 888 Question City Plaza, Seattle, WA 98101, United States
WhatsApp: +1 (206) 555-7890
Website: WHAT.EDU.VN
Don’t miss out on the opportunity to expand your knowledge and get the answers you need. Ask your question now on what.edu.vn!