Deoxyribonucleic acid, also known as DNA, is the fundamental hereditary material in humans and nearly all other living organisms. Discover how this molecule of life functions and why it’s so critical for understanding life itself on WHAT.EDU.VN. Explore its structure, function, and significance with our accessible explanations, perfect for answering all your burning questions about genetic material and hereditary information.
1. Defining Deoxyribonucleic Acid: The Blueprint of Life
Deoxyribonucleic acid (DNA) serves as the instruction manual or blueprint for life. It contains the genetic instructions used in the growth, development, functioning, and reproduction of all known living organisms and many viruses. DNA is a nucleic acid, and along with proteins, lipids and carbohydrates, is one of the four major types of macromolecules that are essential for all known forms of life. It’s crucial for understanding how traits are passed down from one generation to the next. Think of DNA as the software code that dictates how an organism is built and operates. This code is made up of a sequence of smaller units called nucleotides.
1.1. The Structure of DNA: The Double Helix Explained
DNA has a unique structure known as a double helix, often described as a twisted ladder. This structure allows DNA to store a vast amount of information in a compact and stable form. The sides of the ladder are made up of sugar and phosphate molecules, while the rungs are formed by pairs of nitrogenous bases. These bases are adenine (A), guanine (G), cytosine (C), and thymine (T). Adenine always pairs with thymine (A-T), and cytosine always pairs with guanine (C-G).
The double helix structure was famously discovered by James Watson and Francis Crick in 1953, building upon the work of Rosalind Franklin and Maurice Wilkins. Their discovery revolutionized biology and paved the way for understanding the genetic code and how it’s transmitted.
1.2. Where is DNA Found? Nuclear and Mitochondrial DNA
In eukaryotic cells (cells with a nucleus), most DNA is located within the cell nucleus. This is referred to as nuclear DNA. However, a small amount of DNA is also found in the mitochondria, which are organelles responsible for energy production within the cell. This is known as mitochondrial DNA (mtDNA). In prokaryotic cells (cells without a nucleus), such as bacteria, DNA is located in the cytoplasm.
Mitochondrial DNA is unique because it is inherited solely from the mother. This makes it a useful tool for tracing maternal lineages in genetics and evolutionary studies. It’s also involved in certain genetic disorders.
1.3. Chromosomes: Packaging DNA for Efficiency
Within the nucleus, DNA is organized into structures called chromosomes. Humans have 23 pairs of chromosomes, for a total of 46. One set of 23 chromosomes is inherited from each parent. Chromosomes ensure that DNA is properly duplicated and distributed during cell division. They also protect DNA from damage.
Each chromosome is made up of a single, long DNA molecule tightly coiled around proteins called histones. This packaging allows a large amount of DNA to fit within the small space of the cell nucleus.
2. The Building Blocks of DNA: Nucleotides and Base Pairs
Understanding the components of DNA helps clarify how it stores and transmits genetic information. The basic building block of DNA is the nucleotide, which consists of a sugar molecule, a phosphate group, and a nitrogenous base.
2.1. The Four Nitrogenous Bases: A, T, C, and G
As mentioned earlier, DNA contains four nitrogenous bases: adenine (A), thymine (T), cytosine (C), and guanine (G). The sequence of these bases along the DNA molecule determines the genetic code. Each base has a specific chemical structure that allows it to pair with its complementary base. Adenine (A) always pairs with thymine (T), and cytosine (C) always pairs with guanine (G).
2.2. Base Pairing: The Foundation of DNA Replication
The specific pairing of bases (A-T and C-G) is crucial for DNA replication and transcription. This is because each strand of DNA in the double helix acts as a template for creating a new, complementary strand. During replication, the double helix unwinds, and each strand serves as a template for synthesizing a new strand. Enzymes called DNA polymerases facilitate this process, ensuring that the new DNA molecules are identical to the original.
2.3. The Sugar-Phosphate Backbone: Providing Structural Support
The sugar-phosphate backbone forms the structural framework of the DNA molecule. It consists of alternating sugar (deoxyribose) and phosphate groups linked together in a chain. The nitrogenous bases are attached to the sugar molecules, projecting inward toward the center of the double helix. The sugar-phosphate backbone is essential for maintaining the stability and integrity of the DNA molecule.
Alt Text: Illustration depicting the structure of DNA, showcasing the arrangement of base pairs and the sugar-phosphate backbone in the double helix.
3. DNA Replication: Copying the Code of Life
DNA replication is the process by which DNA makes copies of itself. This is essential for cell division, ensuring that each new cell receives a complete and accurate copy of the genetic information.
3.1. The Process of DNA Replication: A Step-by-Step Guide
DNA replication is a complex process involving several enzymes and proteins. Here’s a simplified overview:
- Initiation: Replication begins at specific sites on the DNA molecule called origins of replication.
- Unwinding: The enzyme helicase unwinds the double helix, separating the two strands.
- Primer Synthesis: An enzyme called primase synthesizes short RNA primers that provide a starting point for DNA synthesis.
- DNA Synthesis: DNA polymerase enzymes add nucleotides to the 3′ end of the primer, synthesizing a new DNA strand complementary to the template strand.
- Proofreading: DNA polymerase enzymes proofread the newly synthesized DNA, correcting any errors.
- Termination: Replication continues until the entire DNA molecule has been copied.
3.2. Enzymes Involved in DNA Replication: Key Players
Several enzymes play critical roles in DNA replication:
- Helicase: Unwinds the double helix.
- Primase: Synthesizes RNA primers.
- DNA Polymerase: Adds nucleotides to the growing DNA strand and proofreads the new DNA.
- Ligase: Joins DNA fragments together.
3.3. The Importance of Accurate Replication: Maintaining Genetic Integrity
Accurate DNA replication is essential for maintaining the integrity of the genetic code. Errors during replication can lead to mutations, which can have harmful effects on the cell or organism. DNA polymerase enzymes have proofreading capabilities to minimize errors, but some mutations can still occur.
4. DNA vs. RNA: Key Differences
While both DNA and RNA are nucleic acids and play vital roles in genetics, they have distinct differences in structure and function.
4.1. Structural Differences: Single vs. Double Stranded
DNA is a double-stranded molecule, while RNA is typically single-stranded. DNA contains the sugar deoxyribose, while RNA contains the sugar ribose. DNA uses the base thymine (T), while RNA uses the base uracil (U).
4.2. Functional Differences: Storage vs. Expression of Genetic Information
DNA’s primary function is to store genetic information. RNA, on the other hand, is involved in expressing that information. There are several types of RNA, each with a specific role:
- mRNA (messenger RNA): Carries genetic information from DNA to the ribosomes, where proteins are synthesized.
- tRNA (transfer RNA): Brings amino acids to the ribosomes during protein synthesis.
- rRNA (ribosomal RNA): Forms part of the ribosomes, the cellular machinery responsible for protein synthesis.
4.3. Stability: DNA’s Long-Term Storage vs. RNA’s Short-Term Use
DNA is a more stable molecule than RNA, making it ideal for long-term storage of genetic information. RNA is more prone to degradation and is typically used for short-term purposes, such as protein synthesis.
5. Genes and the Genetic Code: Decoding DNA’s Instructions
A gene is a segment of DNA that contains the instructions for building a specific protein or RNA molecule. The genetic code is the set of rules by which information encoded in genetic material (DNA or RNA) is translated into proteins by living cells.
5.1. What is a Gene? Defining Units of Heredity
A gene is the basic unit of heredity and is responsible for passing traits from parents to offspring. Genes are located on chromosomes and consist of a specific sequence of DNA bases.
5.2. The Genetic Code: Translating DNA into Proteins
The genetic code is based on a three-base code called codons. Each codon specifies a particular amino acid, which is the building block of proteins. There are 64 possible codons, with 61 coding for amino acids and 3 serving as stop signals to terminate protein synthesis.
5.3. Mutations: Alterations in the Genetic Code and Their Effects
Mutations are changes in the DNA sequence that can occur spontaneously or be induced by external factors such as radiation or chemicals. Mutations can have various effects on the organism, ranging from no effect to severe disease. Some mutations can even be beneficial, driving evolution and adaptation.
6. DNA Sequencing: Reading the Genetic Code
DNA sequencing is the process of determining the precise order of nucleotides within a DNA molecule. This technology has revolutionized biology and medicine, allowing scientists to study genes, diagnose diseases, and develop new treatments.
6.1. Methods of DNA Sequencing: From Sanger to Next-Generation Sequencing
The first method of DNA sequencing was developed by Frederick Sanger in the 1970s. Sanger sequencing is a chain-termination method that involves synthesizing DNA strands of different lengths and then separating them based on size. Next-generation sequencing (NGS) technologies have emerged in recent years, allowing for much faster and cheaper sequencing of DNA.
6.2. Applications of DNA Sequencing: Unlocking Genetic Information
DNA sequencing has numerous applications:
- Gene Identification: Identifying genes associated with diseases.
- Diagnosis: Diagnosing genetic disorders and infectious diseases.
- Personalized Medicine: Tailoring medical treatments based on an individual’s genetic makeup.
- Forensics: Identifying individuals in criminal investigations.
- Evolutionary Biology: Studying the evolutionary relationships between different organisms.
6.3. Ethical Considerations in DNA Sequencing: Privacy and Data Security
DNA sequencing raises ethical concerns regarding privacy and data security. Genetic information is highly personal and can be used to discriminate against individuals. It’s crucial to have regulations and safeguards in place to protect genetic data and prevent misuse.
7. DNA Technology: Manipulating the Genetic Code
DNA technology involves the manipulation of DNA for various purposes, such as creating genetically modified organisms, developing gene therapies, and producing recombinant proteins.
7.1. Recombinant DNA Technology: Combining DNA from Different Sources
Recombinant DNA technology involves combining DNA from different sources to create new DNA molecules. This technology is used to produce recombinant proteins, such as insulin and growth hormone, which are used to treat diseases.
7.2. Gene Editing: Modifying Genes with Precision
Gene editing technologies, such as CRISPR-Cas9, allow scientists to precisely modify genes within living organisms. This technology has the potential to cure genetic diseases, create disease-resistant crops, and develop new therapies for cancer.
7.3. Applications of DNA Technology: Transforming Medicine and Agriculture
DNA technology has transformed medicine and agriculture:
- Gene Therapy: Treating genetic diseases by replacing faulty genes with healthy ones.
- Genetically Modified Organisms (GMOs): Creating crops that are resistant to pests, herbicides, and harsh environmental conditions.
- Drug Development: Developing new drugs and therapies for various diseases.
- Diagnostics: Developing new diagnostic tools for detecting diseases.
8. DNA and Heredity: Passing Traits from Parents to Offspring
DNA is the basis of heredity, the process by which traits are passed from parents to offspring. During sexual reproduction, each parent contributes half of their DNA to their offspring.
8.1. How DNA Determines Traits: Genes and Phenotypes
Genes, which are segments of DNA, determine an individual’s traits, or phenotype. Some traits are determined by a single gene, while others are determined by multiple genes interacting with each other and the environment.
8.2. Inheritance Patterns: Dominant and Recessive Genes
Inheritance patterns depend on whether a gene is dominant or recessive. A dominant gene will express its trait even if only one copy is present, while a recessive gene will only express its trait if two copies are present.
8.3. Genetic Disorders: When DNA Goes Wrong
Genetic disorders are caused by mutations in DNA that disrupt normal gene function. These disorders can be inherited from parents or occur spontaneously. Examples of genetic disorders include cystic fibrosis, sickle cell anemia, and Huntington’s disease.
9. The Human Genome Project: Mapping the Entire Human DNA
The Human Genome Project was an international scientific research project with the primary goal of determining the complete sequence of human DNA and identifying all of the genes within the human genome.
9.1. Goals and Achievements of the Human Genome Project
The Human Genome Project achieved its goals of sequencing the entire human genome and identifying all of the genes. This project has had a profound impact on biology and medicine, providing a foundation for understanding human health and disease.
9.2. Impact on Medicine and Research: New Avenues for Discovery
The Human Genome Project has opened up new avenues for discovery in medicine and research:
- Personalized Medicine: Tailoring medical treatments based on an individual’s genetic makeup.
- Gene Therapy: Treating genetic diseases by replacing faulty genes with healthy ones.
- Drug Development: Developing new drugs and therapies for various diseases.
- Diagnostics: Developing new diagnostic tools for detecting diseases.
9.3. Future Directions in Genomics: Beyond the Human Genome
The field of genomics continues to advance rapidly, with new technologies and approaches being developed. Future directions in genomics include:
- Functional Genomics: Understanding the functions of genes and how they interact with each other.
- Comparative Genomics: Comparing the genomes of different organisms to understand evolution and identify conserved genes.
- Metagenomics: Studying the genetic material recovered directly from environmental samples.
10. Frequently Asked Questions About Deoxyribonucleic Acid (DNA)
Here are some frequently asked questions about DNA:
Question | Answer |
---|---|
What is the main function of DNA? | The main function of DNA is to store and transmit genetic information, providing the instructions for building and maintaining an organism. |
How does DNA determine our traits? | DNA determines our traits through genes, which are segments of DNA that code for specific proteins. These proteins carry out various functions in the body, influencing our physical characteristics, such as eye color and height, as well as our susceptibility to certain diseases. |
What is the difference between a gene and a chromosome? | A gene is a specific segment of DNA that codes for a particular trait or function, while a chromosome is a larger structure made up of DNA tightly coiled around proteins called histones. Chromosomes contain many genes. |
What are the ethical concerns related to DNA technology? | Ethical concerns related to DNA technology include privacy and data security, as genetic information is highly personal and can be used to discriminate against individuals. There are also concerns about the potential misuse of gene editing technologies and the long-term effects of genetically modified organisms (GMOs). |
Where can I learn more about DNA and genetics? | You can learn more about DNA and genetics from various resources, including textbooks, scientific journals, reputable websites, and educational institutions. Many universities and research institutions offer courses and programs in genetics and related fields. WHAT.EDU.VN offers a platform to ask any questions you have and receive clear, concise answers. |
How accurate is DNA replication? | DNA replication is a highly accurate process, thanks to the proofreading capabilities of DNA polymerase enzymes. However, errors can still occur, leading to mutations. The error rate is estimated to be about one in every 10 billion base pairs. |
What is the role of DNA in evolution? | DNA plays a crucial role in evolution by providing the raw material for genetic variation. Mutations in DNA can lead to new traits that may be beneficial to an organism’s survival and reproduction. Over time, these beneficial traits can become more common in a population, leading to evolutionary change. |
How is DNA used in forensics? | DNA is used in forensics to identify individuals in criminal investigations. DNA samples collected from crime scenes can be compared to DNA samples from suspects to determine if there is a match. DNA evidence is highly reliable and is often used to convict criminals. |
Can DNA be used to predict future health risks? | DNA can be used to predict future health risks by identifying genes associated with certain diseases. However, it’s important to note that genetics is only one factor that influences health. Lifestyle factors, such as diet and exercise, also play a significant role. |
Is it possible to repair damaged DNA? | Yes, cells have various mechanisms for repairing damaged DNA. These mechanisms include DNA repair enzymes that can identify and correct errors in the DNA sequence. However, if DNA damage is too extensive, it may not be possible to repair it, which can lead to mutations and disease. |
What are some current research areas in DNA and genetics? | Some current research areas in DNA and genetics include personalized medicine, gene therapy, CRISPR-Cas9 gene editing, synthetic biology, and metagenomics. These areas of research hold promise for improving human health, developing new technologies, and understanding the natural world. |
How does the study of DNA contribute to our understanding of ancestry? | DNA analysis can reveal ancestral origins by comparing genetic markers across different populations and geographic regions. This helps trace migration patterns and understand human history. |
What are the differences in DNA among different species of organisms? | DNA varies significantly between species in terms of the number of chromosomes, gene sequences, and overall genome size. These differences reflect the unique evolutionary paths and adaptations of each species. |
How does DNA contribute to the aging process? | DNA damage accumulates over time, contributing to the aging process. This damage can impair cell function and lead to age-related diseases. Research into DNA repair mechanisms and anti-aging interventions aims to mitigate these effects. |
How can I contribute to DNA and genetics research? | You can contribute to DNA and genetics research by participating in citizen science projects, donating to research organizations, and staying informed about the latest scientific findings. Supporting policies that promote scientific research and education is also crucial. |
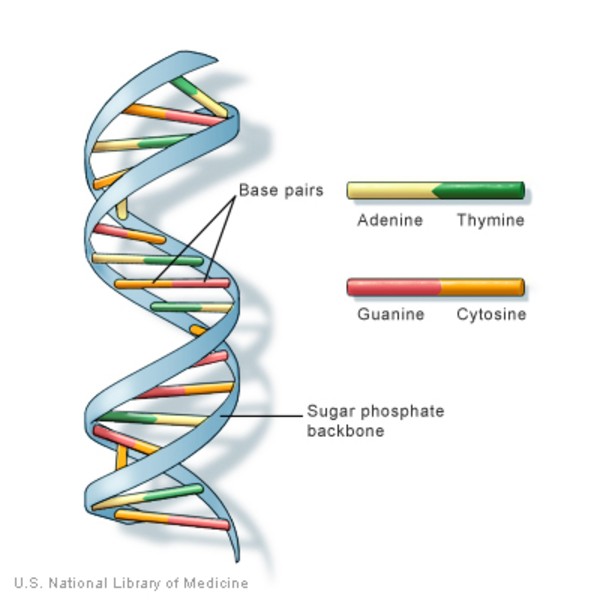
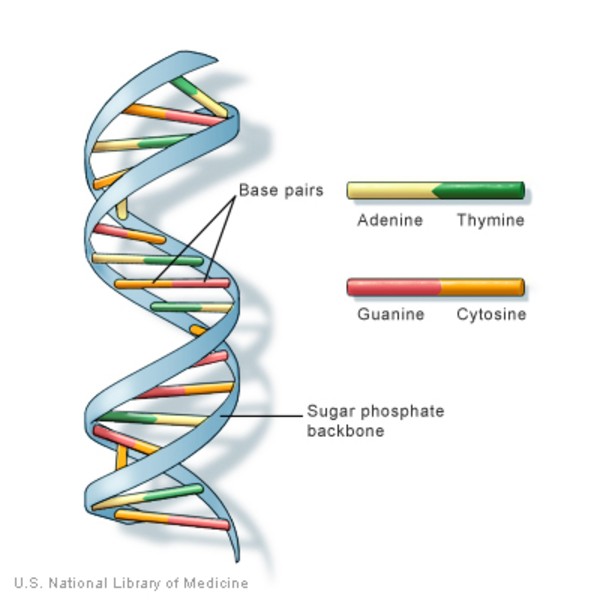
11. The Future of DNA Research and Technology
DNA research and technology continue to evolve at a rapid pace, promising to revolutionize medicine, agriculture, and other fields.
11.1. Emerging Technologies in DNA Analysis
Emerging technologies in DNA analysis include nanopore sequencing, which allows for real-time sequencing of long DNA molecules, and single-cell genomics, which enables the study of the genetic material of individual cells.
11.2. Potential Breakthroughs in Disease Treatment
Potential breakthroughs in disease treatment include the development of new gene therapies for genetic disorders, personalized cancer treatments based on an individual’s genetic makeup, and new vaccines and antiviral drugs based on DNA technology.
11.3. Ethical and Societal Implications of Future DNA Technologies
As DNA technologies become more powerful, it’s important to consider the ethical and societal implications. These include issues such as genetic privacy, access to genetic testing and therapies, and the potential for genetic discrimination. Open and informed discussions are needed to ensure that these technologies are used responsibly and for the benefit of all.
12. Conclusion: Embracing the Power of Deoxyribonucleic Acid
Deoxyribonucleic acid (DNA) is the fundamental molecule of life, carrying the genetic instructions that make each organism unique. Understanding DNA is essential for understanding biology, medicine, and the world around us. From its elegant double helix structure to its complex mechanisms of replication and expression, DNA continues to fascinate and inspire scientists and researchers. As DNA technologies continue to advance, they offer the potential to transform medicine, agriculture, and other fields, improving human health and well-being.
Have questions about deoxyribonucleic acid, genetics, or anything else? Don’t struggle to find answers on your own. At WHAT.EDU.VN, we provide a platform where you can ask any question and receive clear, concise answers from experts in the field, all for free. Whether you’re a student, a professional, or simply curious about the world around you, we’re here to help you learn and grow. Visit us today at what.edu.vn or contact us at 888 Question City Plaza, Seattle, WA 98101, United States or Whatsapp: +1 (206) 555-7890. Let’s unlock the secrets of the universe together.
Alt Text: Detailed illustration of DNA’s double helix structure, highlighting the nucleotide components, sugar-phosphate backbone, and pairing of bases.