What Is The Monomer Of Proteins? Delve into the fascinating world of proteins with WHAT.EDU.VN and discover the fundamental building blocks that make up these essential macromolecules. We’ll explore the monomer units, their structure, and how they contribute to protein diversity and function.
Proteins are the workhorses of the cell, performing a vast array of functions, from catalyzing biochemical reactions to providing structural support. Understanding their basic building blocks is crucial to understanding their complex roles. Want to unravel the mysteries of protein structure and function? Ask your questions on WHAT.EDU.VN and get free answers today. Uncover the critical role of amino acids, peptide bonds, and polypeptide chains in shaping protein structure.
1. Understanding Biological Macromolecules
Biological macromolecules are essential for life and are constructed from smaller organic molecules. These large molecules perform a wide range of functions within cells and organisms.
1.1. Four Major Classes
There are four primary classes of biological macromolecules:
- Carbohydrates
- Lipids
- Proteins
- Nucleic acids
These macromolecules are crucial components of cells, each with specific roles. Together, they constitute the majority of a cell’s dry mass. All biological macromolecules are organic, meaning they contain carbon, along with hydrogen, oxygen, nitrogen, and other trace elements.
1.2. Monomers and Polymers
Most macromolecules are assembled from single subunits known as monomers. These monomers link together through covalent bonds to form larger structures called polymers. During this process, monomers release water molecules as byproducts. This reaction is called dehydration synthesis.
Dehydration synthesis joins monomers by removing a water molecule, creating a polymer.
1.2.1. Dehydration Synthesis
Dehydration synthesis, also known as condensation, means “to make while losing water.” In this reaction, the hydrogen atom of one monomer combines with the hydroxyl group of another, releasing a water molecule (H2O). Simultaneously, the monomers share electrons and form a covalent bond. As more monomers join, this chain of repeating units forms a polymer. Different types of monomers can combine in various configurations, resulting in diverse macromolecules.
1.2.2. Hydrolysis Reactions
Polymers can be broken down into monomers through a process called hydrolysis, which means “to split water.” During hydrolysis, water is added to break the bonds between monomers. The polymer is split into two components: one part gains a hydrogen atom (H+), and the other gains a hydroxyl molecule (OH-) from the water molecule.
Hydrolysis uses water to break the bonds in a polymer, separating it into individual monomers.
1.3. Enzymes and Macromolecule Breakdown
Dehydration and hydrolysis reactions are catalyzed by specific enzymes, which speed up the reactions. Dehydration reactions require energy to form new bonds, while hydrolysis reactions release energy when breaking bonds. These reactions are similar across most macromolecules, but each monomer and polymer reaction is specific to its class.
For example, food is hydrolyzed into smaller molecules by catalytic enzymes in the digestive system, allowing for easy absorption of nutrients by cells in the intestine. Each macromolecule is broken down by a specific enzyme. Carbohydrates are broken down by amylase, sucrase, lactase, or maltase. Proteins are broken down by pepsin and peptidase, along with hydrochloric acid. Lipids are broken down by lipases. The breakdown of these macromolecules provides energy for cellular activities.
Want to know more about enzymes and their roles in breaking down macromolecules? Visit WHAT.EDU.VN for quick, free answers.
2. Proteins: Structure, Types, and Functions
Proteins are among the most abundant organic molecules in living systems and have the most diverse range of functions compared to other macromolecules. They are polymers of amino acids arranged in a linear sequence. Proteins can be structural, regulatory, contractile, or protective. They serve in transport, storage, or membranes and can also act as toxins or enzymes. Each cell in a living system contains thousands of proteins, each with a unique function and structure.
2.1. Diverse Functions of Proteins
Proteins perform a multitude of functions essential for life. Here are some key roles they play:
- Structural Proteins: Provide support and shape to cells and tissues. Examples include keratin in skin and collagen in connective tissue.
- Regulatory Proteins: Control cell processes and gene expression.
- Contractile Proteins: Enable movement. Actin and myosin are examples found in muscles.
- Protective Proteins: Defend the body against foreign invaders. Antibodies are a prime example.
- Transport Proteins: Carry substances across cell membranes or throughout the body. Hemoglobin transports oxygen in the blood.
- Storage Proteins: Store nutrients and energy.
- Membrane Proteins: Function as receptors, channels, and pumps in cell membranes.
- Enzymes: Catalyze biochemical reactions.
2.2 Protein Types and Functions
The table below provides examples of protein types and their corresponding functions:
Type | Examples | Functions |
---|---|---|
Digestive Enzymes | Amylase, lipase, pepsin, trypsin | Help digest food by breaking down nutrients into monomeric units |
Transport | Hemoglobin, albumin | Carry substances in the blood or lymph throughout the body |
Structural | Actin, tubulin, keratin | Construct different structures, like the cytoskeleton |
Hormones | Insulin, thyroxine | Coordinate the activity of different body systems |
Defense | Immunoglobulins | Protect the body from foreign pathogens |
Contractile | Actin, myosin | Effect muscle contraction |
Storage | Legume storage proteins, albumin | Provide nourishment in early development of the embryo and the seedling |
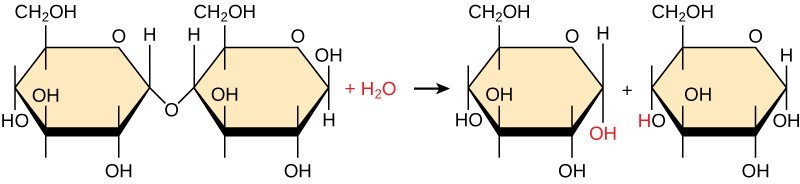
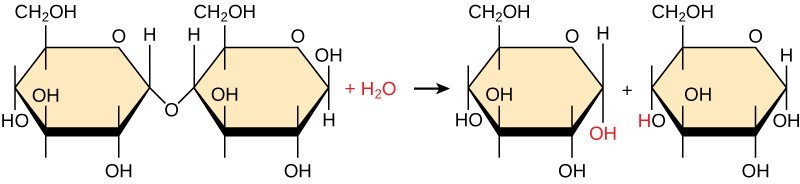
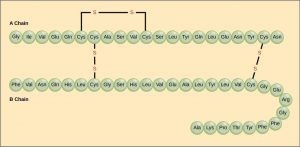
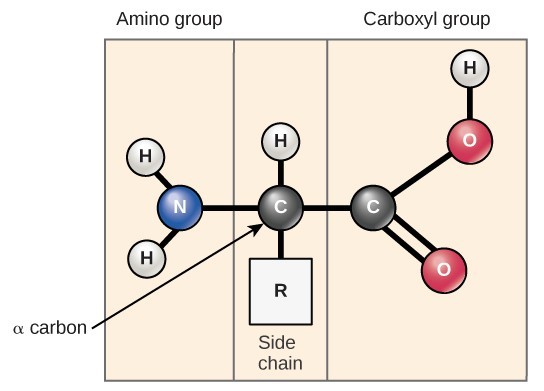
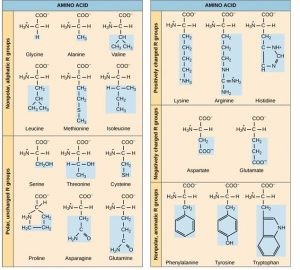
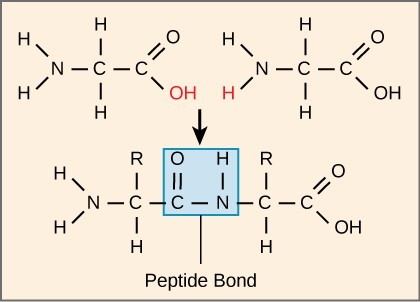
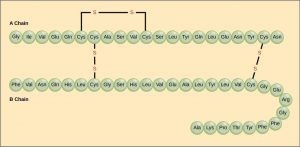
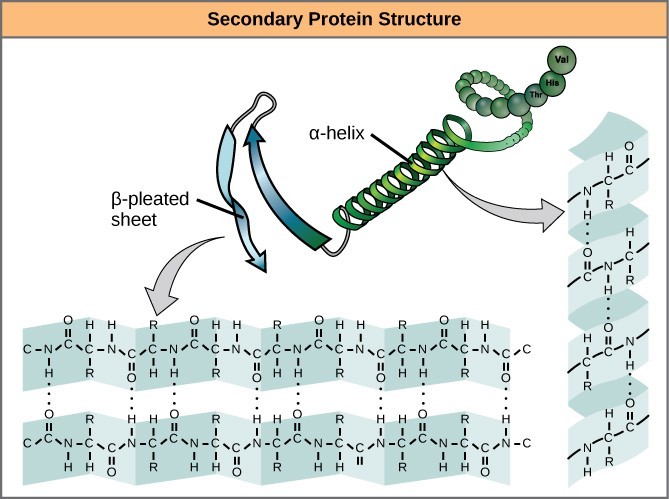
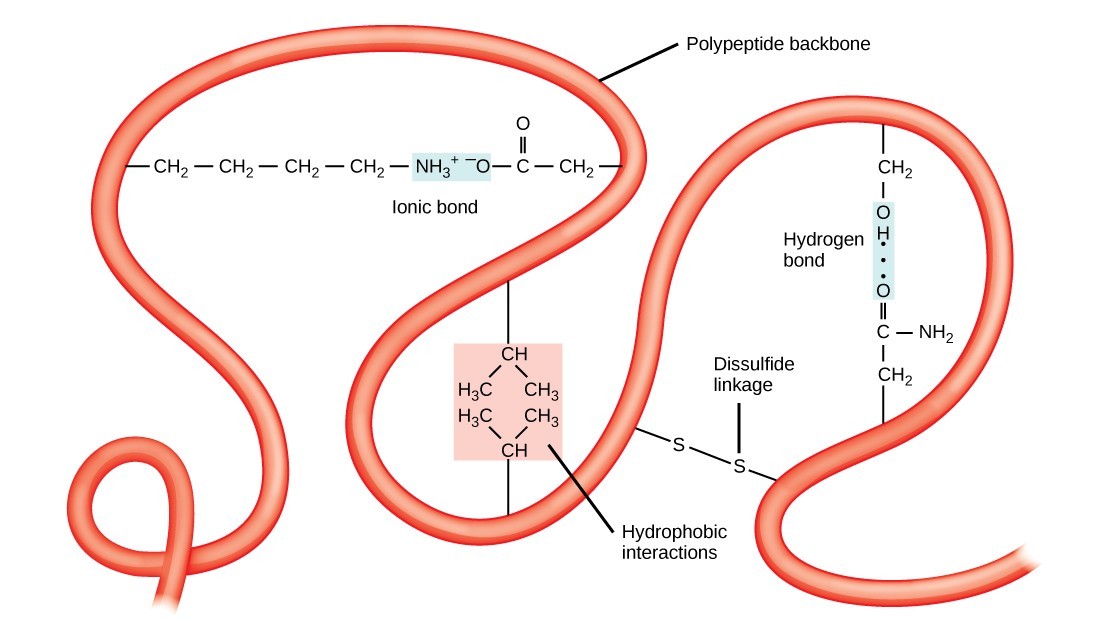
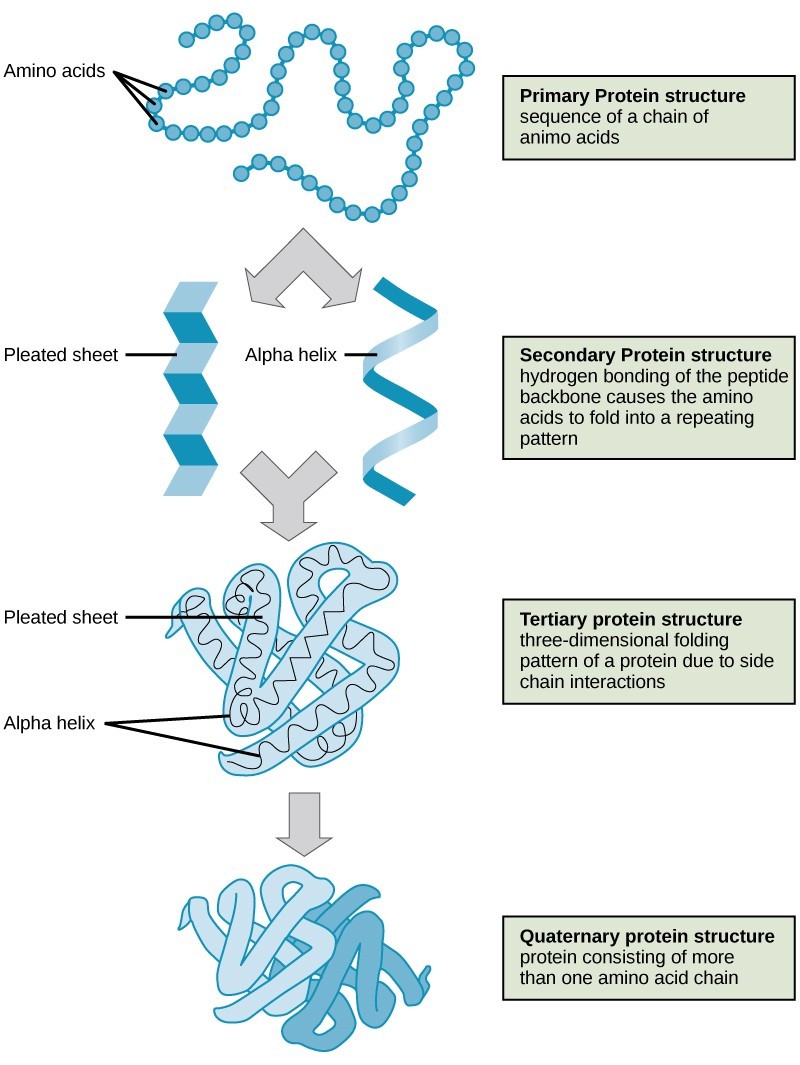
2.3. Protein Shape and Denaturation
The shape of a protein is critical to its function and is maintained by various chemical bonds. Changes in temperature, pH, or exposure to chemicals can lead to permanent changes in the protein’s shape, resulting in a loss of function known as denaturation.
Proteins like hemoglobin have globular shapes, while collagen has a fibrous structure.
3. Amino Acids: The Monomers of Proteins
Amino acids are the monomers that make up proteins. All proteins are constructed from different arrangements of the same 20 amino acids.
3.1. Basic Structure of Amino Acids
Each amino acid has a central carbon atom bonded to:
- An amino group (NH2)
- A carboxyl group (COOH)
- A hydrogen atom
- A variable R group (side chain)
The name “amino acid” comes from the presence of the amino group and the acidic carboxyl group.
The structure of an amino acid includes a central carbon bonded to an amino group, a carboxyl group, a hydrogen atom, and an R group.
3.2. Essential and Non-Essential Amino Acids
The same 20 common amino acids are present in proteins from all species of life. Ten of these are considered essential amino acids in humans because the human body cannot produce them and they must be obtained from the diet.
3.3. R Groups and Amino Acid Properties
Each amino acid differs only in its R group (side chain). The chemical nature of the R group determines the nature of the amino acid, such as whether it is acidic, basic, polar, or nonpolar.
- Nonpolar (Hydrophobic) Amino Acids: Examples include valine, methionine, and alanine.
- Polar (Hydrophilic) Amino Acids: Examples include serine, threonine, and cysteine.
- Positively Charged (Basic) Amino Acids: Examples include lysine and arginine.
- Negatively Charged (Acidic) Amino Acids: Examples include aspartate and glutamate.
The R groups of amino acids determine their chemical properties, influencing protein structure and function.
3.4. Polypeptides and Peptide Bonds
Amino acids are linked together into linear chains called polypeptides. While the terms polypeptide and protein are sometimes used interchangeably, a polypeptide is technically a polymer of amino acids, whereas the term protein refers to a polypeptide or polypeptides that have a distinct shape and a unique function. The sequence and number of amino acids determine the protein’s shape, size, and function. After protein synthesis (translation), most proteins are modified. Only after these modifications is the protein completely functional.
3.5. Peptide Backbone
Each polypeptide has an N terminal, with a free amino group, and a C terminal, with a free carboxyl group. Amino acids are attached to other amino acids by covalent bonds known as peptide bonds, which are formed by dehydration synthesis reactions. The carboxyl group of one amino acid and the amino group of the incoming amino acid combine, releasing a molecule of water and forming a peptide bond. Additional amino acids are always added to the C terminus until the chain is complete. The resulting polypeptide chain has a peptide backbone (or carbon-nitrogen backbone) that is identical for all proteins, with the variable R groups extending off of the backbone. Polypeptides differ from each other only in the order of the R groups.
Peptide bond formation involves the removal of a water molecule, linking the carboxyl group of one amino acid to the amino group of another.
4. Protein Structure: Primary, Secondary, Tertiary, and Quaternary
The structure of a protein is critical to its function. For example, an enzyme can bind to a specific substrate at a site known as the active site. If this active site is altered because of local changes or changes in overall protein structure, the enzyme may be unable to bind to the substrate. Understanding the four levels of protein structure—primary, secondary, tertiary, and quaternary—is essential for understanding how proteins achieve their final shape or conformation.
4.1. Primary Structure
The unique sequence of amino acids in a polypeptide chain is its primary structure. For example, the pancreatic hormone insulin has two polypeptide chains, A and B, which are linked together by disulfide bonds. The primary structure of each chain is indicated by three-letter abbreviations that represent the names and order of the amino acids. The N terminal amino acid of the A chain is glycine, whereas the C terminal amino acid is asparagine. The sequences of amino acids in the A and B chains are unique to insulin.
Insulin’s primary structure includes two chains, A and B, connected by disulfide bonds.
4.2. Secondary Structure
The local folding of the polypeptide in some regions gives rise to the secondary structure of the protein. The most common secondary structures are the α-helix and β-pleated sheet. Both structures are formed by hydrogen bonds between parts of the peptide backbone of the polypeptide. Specifically, the oxygen atom in the carbonyl group in one amino acid interacts with another amino acid that is four amino acids farther along the chain.
Alpha-helices and beta-pleated sheets are stabilized by hydrogen bonds along the peptide backbone.
4.3. Tertiary Structure
The unique three-dimensional structure of a polypeptide is its tertiary structure. This structure is primarily due to interactions among R groups. For example, R groups with like charges are repelled by each other, and those with unlike charges are attracted to each other via ionic bonds. When protein folding takes place in a watery environment, such as that found inside cells, the hydrophobic R groups of nonpolar amino acids lay in the interior of the protein, while the hydrophilic R groups face out. Hydrophobic R groups also interact with each other through van der Waals forces. Interaction between cysteine side chains forms disulfide linkages, which are the only covalent bond formed during protein folding. All of these interactions determine the final three-dimensional shape of the protein. When a protein loses its three-dimensional shape, it may no longer be functional.
Tertiary structure is stabilized by interactions like hydrophobic interactions, ionic bonds, hydrogen bonds, and disulfide linkages.
4.4. Quaternary Structure
In nature, some proteins are formed from several separate polypeptides, known as subunits. The interaction of these subunits forms the quaternary structure of a protein. Weak interactions between the subunits help to stabilize the overall structure. For example, silk is a fibrous protein that results from hydrogen bonding between different chains.
The four levels of protein structure determine a protein’s final shape and function.
4.5. Denaturation and Protein Folding
If a protein is subject to changes in temperature, pH, or exposure to chemicals, it may lose its shape, a process called denaturation. The primary structure of the protein is not changed by denaturation, but some or all of the folding is lost. Denaturation is often reversible, allowing the protein to resume its function. Sometimes denaturation is irreversible, leading to permanent loss of function. One example of irreversible protein denaturation is cooking an egg white. Different proteins denature under different conditions. For example, bacteria from hot springs have proteins that function at temperatures close to boiling. Although stomach acid denatures proteins as part of digestion, the digestive enzymes of the stomach retain their activity under these conditions.
Correct folding of proteins is critical to their function. Although some proteins fold automatically, recent research has discovered that some proteins receive assistance in folding from protein helpers known as chaperones.
5. The Evolutionary Significance of Cytochrome c
Cytochrome c is a protein that is important for cellular respiration. Sequencing the cytochrome c protein from different species has shown that there is a considerable amount of amino acid sequence homology among different species. Evolutionary kinship can be assessed by measuring the similarities or differences among various species’ DNA or protein sequences.
Human cytochrome c contains 104 amino acids. 37 of these amino acids appear in the same position in samples of cytochrome c from a variety of different species. Human and chimpanzee cytochrome c is identical, and human and rhesus monkey cytochrome c differ in only one amino acid. Surprisingly, human and yeast cytochrome c also differ in just one amino acid.
6. Common Questions About Protein Monomers
To further enhance your understanding, here are some frequently asked questions about the monomer of proteins:
Question | Answer |
---|---|
What is the basic building block of a protein? | The basic building block of a protein is an amino acid. |
How many different types of amino acids are there? | There are 20 different types of amino acids commonly found in proteins. |
What part of the amino acid makes each one unique? | The R group, or side chain, makes each amino acid unique, determining its chemical properties. |
What type of bond links amino acids together? | Amino acids are linked together by peptide bonds, which are formed through dehydration synthesis. |
What is a polypeptide? | A polypeptide is a chain of amino acids linked together by peptide bonds. |
How does the sequence of amino acids affect protein function? | The sequence of amino acids determines the protein’s shape, which is crucial for its function. Even a single amino acid change can affect the protein’s activity. |
What are essential amino acids? | Essential amino acids are those that the human body cannot synthesize and must be obtained from the diet. |
How are proteins broken down into amino acids? | Proteins are broken down into amino acids through hydrolysis, a process that involves the addition of water to break the peptide bonds. |
What role do enzymes play in protein synthesis and breakdown? | Enzymes catalyze both the synthesis of proteins from amino acids and the breakdown of proteins into amino acids, speeding up these essential biological processes. |
Why is protein folding important? | Protein folding is crucial because the three-dimensional shape of a protein determines its function. Incorrect folding can lead to non-functional or harmful proteins. |
7. Need More Answers?
Understanding the monomers of proteins is essential for grasping the complexities of biochemistry and molecular biology. The amino acids, with their unique structures and properties, are the foundation upon which all proteins are built.
Do you have more questions about proteins, amino acids, or any other topic? Don’t hesitate to ask! At WHAT.EDU.VN, we provide a free platform for you to get your questions answered quickly and accurately.
Address: 888 Question City Plaza, Seattle, WA 98101, United States
WhatsApp: +1 (206) 555-7890
Website: WHAT.EDU.VN
8. Call to Action
Are you struggling to find quick and free answers to your questions? Do you need a reliable platform to connect with knowledgeable individuals? Visit WHAT.EDU.VN today and experience the convenience of asking any question and receiving timely, accurate responses. Join our community and get the answers you need, absolutely free!
At WHAT.EDU.VN, we understand the challenges of finding trustworthy information. That’s why we’ve created a user-friendly platform where you can ask questions and receive answers from experts and community members alike. Whether you’re a student, a professional, or simply curious, WHAT.EDU.VN is here to help.
Don’t let your questions go unanswered. Visit what.edu.vn now and discover the ease of getting free, reliable answers. We’re here to help you explore, learn, and grow. Join our community today!